- Ideas:
- Recent results:
For information on possible PhD projects in this group, please contact Chris Ford.
SAW quantum computation
The idea is to use the spin of the single electrons trapped in SAW potential minima as qubits:
- A single electron is trapped in each SAW potential minimum and is transported through a depleted 1D channel
- High-frequency qubit operations can be made by patterned surface gates/nanomagnets laid out on the chip
- A number of identical operations are repeated at the SAW frequency
- A flying qubit scheme on chip
- A scope for quantum information transfer to different qubit schemes (photon/static quantum dots)
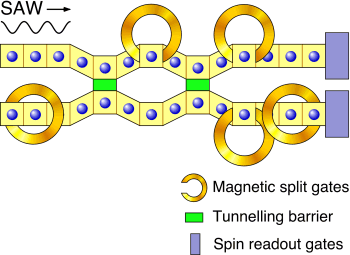
For more information see: Barnes et al., Phys. Rev. B, 62, 8410 (2000).
Quantum information transfer
Qubit states can be transferred to different types of qubits via entanglement. Entanglement between flying and static qubits is especially important for the storage and long-range communication of quantum information.
SAW quantum dot to static quantum dot
- An electron in SAW dot is trapped into a static dot, and is entangled with an electron in another static dot.

SAW quantum dot to photon
- Electrons are transported into p-type region, where they recombine with holes to emit photons.
- The spin information of the electrons are transferred into the circular polarisation of the photons.
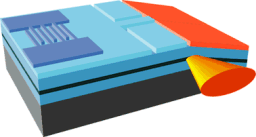
Playing ping-pong with single electrons
A SAW pulse can be used to take an individual electron from one isolated quantum dot to another (from a dot at bottom right in the above figure, to one at top left), and then to send it back again. The process can be repeated without error up to 60 times. As described above, this may be useful for transferring quantum information between a quantum processor and its memory, or between parts of the same processor, using the electron's spin as a qubit. By dropping the electron into a region of holes, a single photon may be generated, either for readout of the electron's spin, or for use as a polarised photon, or even as a photon qubit.

For a more detailed description of this experiment, click here or contact Chris Ford. This work was published in Nature: ‘On-demand single-electron transfer between distant quantum dots’, R. P. G. McNeil et al., Nature 477, 439 (2011).
Interaction of SAWs with a static quantum dot
- A SAW pulse can be used to populate/depopulate an isolated quantum dot.
- A quantum dot is isolated from reservoirs by large barrier potentials.
- An empty (or occupied) state is set below (or above) the Fermi energy.
- Due to large barrier potentials, the dot stays in this non-equilibrium charge state for ~ 100 sec.
- When a SAW pulse is sent through the dot, the potential modulation of the barrier forces the dot into charge equilibrium, populating (or depopulating) the dot by one electron.
- This method can be used to transfer an electron between a SAW dynamic dot and a gate-defined static dot.
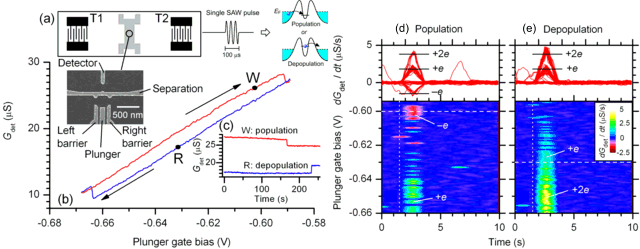
For more information see: Kataoka et al., Phys. Rev. Lett., 98, 046801 (2007).
Coherent time evolution of a single-electron wave-function
Observation of coherent single-electron dynamics is severely limited by experimental bandwidth. We have developed a method to overcome this using moving quantum dots defined by surface acoustic waves. Each dot holds a single electron, and travels through a static potential landscape. When the dot passes abruptly between regions of different confinement, the electron is excited into a superposition of states, and oscillates unitarily from side to side. We detect these oscillations by using a weak, repeated measurement of the current across a tunnel barrier, and find close agreement with simulations.
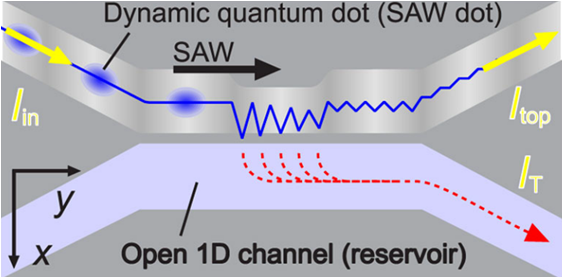
For more information see: Kataoka et al., Phys. Rev. Lett., 102, 156801 (2009), or contact Chris Ford.
Tunnelling behaviour in SAW-defined dynamic quantum dots
- The electrons in a SAW are confined to dynamic quantum dots (zero-dimensional electronic systems).
- By creating devices with tunnelling barriers alongside the SAW channel, we can probe the quantum states of the dot.
- We observe high-frequency quantum behaviour in the form of coherent charge oscillations, and hope to use these devices for spin-charge conversion.

For more information see: Astley et al., Phys. Rev. Lett. 99, 156802 (2007), Astley et al., Physica E, 40, 1017 (2008), or contact Chris Ford.
Investigation of SAW reflections
We have shown that the principal sources of "noise" in the frequency dependence of the acousto-electric current are caused by SAW reflections:
- Reflections come from the edge of the chip and the unused transducer.
- We developed pulse-modulation techniques that modify the effects of different reflections, so we can characterise individual reflection paths and remove them from the experiment.
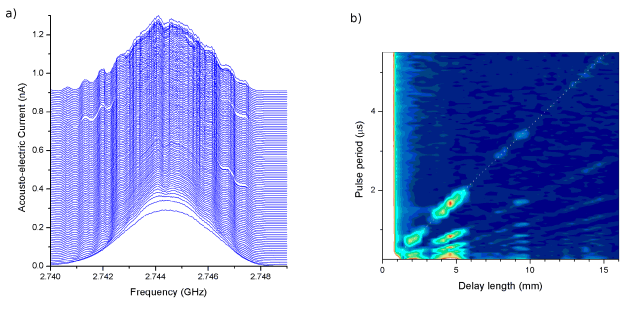
For more information see: Astley et al., Appl. Phys. Lett., 89, 132102 (2006), and Astley et al., Phys. Rev. B, 74, 193302 (2006), or contact Chris Ford.
Numerical modelling of SAW devices
- Electrostatic modelling is used to allow numerical simulation of the experimental devices.
- The time-dependent Schrodinger equation is solved numerically to determine the evolution of an electron in a dynamic quantum dot.

For more information contact Chris Ford and see Kataoka et al., Phys. Rev. Lett., 102, 156801 (2009).